Micromedicine: Using microbubbles and ultrasound for localized drug delivery, treatment and prevention strategies
Engineers from San Diego State University and University of Nebraska-Lincoln collaborate on federally funded research to advance the medical field
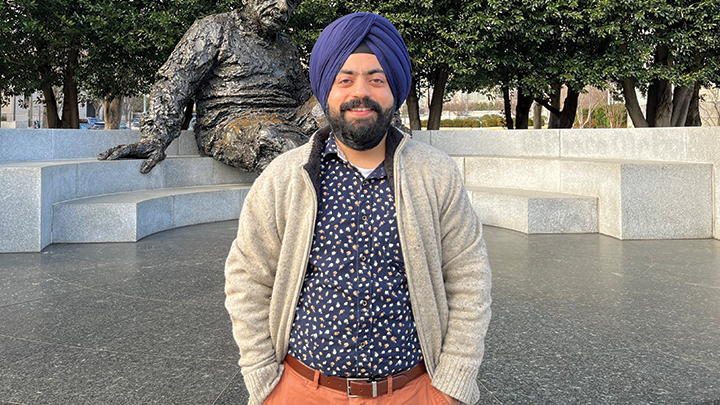
Computer simulations at San Diego State University seek to advance the possible use of microbubbles to deliver drugs into patients, seen as a future treatment for conditions in the brain, heart and other hard-to-reach areas that currently require invasive surgery.
The National Science Foundation-funded joint research project, conducted by San Diego State University Mechanical Engineering Professor Amneet Bhalla and University of Nebraska-Lincoln (UNL) Mechanical and Materials Engineering Professor Nitesh Nama, will develop and perform advanced computer simulations to study the fundamental physical mechanisms that dictate the motion of microbubbles in blood vessels and identify ways to control and tune this motion.
Less than a hundredth of a millimeter in diameter, microbubbles would allow health practitioners to permeate barriers in the human body for diagnosis or treatment, using pressure waves from ultrasound probes.
“There are parts of the human body like the brain, very complex parts that are not accessible. You basically have to cut open the person to do a surgery. So, these micro-swimmers would allow you to conduct non-invasive operations,” Nama said.
“It would not only address mortality but also morbidity, by improving the quality of life for the patient.”
The targeted drug delivery would be much more effective in treating certain diseases. For cancer patients, the technology could someday replace high-dose chemotherapy pills that enter the entire bloodstream. The microbubbles offer a more localized approach that would reduce harmful drug side effects and the need for invasive procedures.
The tiny bubbles would either drop off critical medicine to perform various tasks like clearing blood clots or report information to the doctors through imaging.
Biocompatibility
The researchers will also investigate the fluid mechanics of microbubbles in the bloodstream to assess the viability of using the technique within narrow blood vessels.
“Using physics-based computer simulations, we can investigate emerging diagnostic and preventive medical techniques that are deemed too novel or risky for clinical trials,” said Bhalla.
“Without performing risky animal or human experiments, computer simulations can inform us whether doctors can deliver drug-containing microbubbles through narrow blood vessel passages using biocompatible acoustic power.”
If the theory is validated in the computer model, it will enable more detailed research to be conducted for use in cancer treatment, non-invasive brain surgery, and stroke prevention.
A deeper understanding of fluid mechanics in the human body would significantly improve medical practice, enabling more effective treatments and reducing the need for intensive surgeries and harmful medication side effects.
The findings could also lead to more cutting-edge methods of micromedicine such as using nanorobotics to act as microsurgeons inside the blood vessels, alleviating the need for some highly invasive procedures.
As the microbubble and nanobot technology becomes reality, Nama said, “It would be tremendously helpful in improving our treatment strategies for diseases where we don’t have a solution right now.”
The readily available ultrasound technology works by using acoustics to generate pressure fluctuations in the body, sending waves that reflect as images on the computer screen.
The idea is similar for transporting the micro objects – using an ultrasound probe that vibrates at a very high frequency to create a pressure difference in the blood as a means of delivering the objects to the target location.
“Once the bubbles are in the body, flowing in the veins or in the arteries, they encounter a tremendous amount of resistance from pulsatile blood flow and friction of the vessel walls. So, we are studying how you actually make sure they propel in a controlled manner and reach their destination,” said Bhalla.
The engineers will consider various factors in their investigations such as age, blood pressure and flow, vein and artery texture, and shape to ensure the safety of using this technique in the human body.
“The use of drug-carrying bubbles alleviates the concern of biocompatibility and biosafety,” Bhalla said.
Education and collaboration
The research will train Ph.D. students in sophisticated math and computational modeling using supercomputers that will provide them with opportunities to execute large-scale simulations and analyze results.
“Other researchers have tested this method experimentally in relatively simple microchannels, but we still do not understand the relationship between the applied acoustic waves and the bubble motion,” said Bhalla.
Additionally, through this research project, Bhalla will work with underrepresented students from San Diego County high schools to educate them about mathematical modeling and further their exposure to the STEM field.
Students will have the opportunity to gain hands-on experience, while the engineers inspect the way the bubbles will behave within the bloodstream.
Furthermore, through the UNL Osher Lifelong Learning Institute, Nama will create educational modules to inform the general public about the ongoing project and its findings.
When the modeling concludes, the team will collaborate with Assistant Professor of Acoustic Robotics Daniel Ahmed at ETH Zurich using their newfound data to run advanced microfluidic modeling experiments that mimic human blood vessels.
In parallel, Bhalla and Nama are also collaborating with SDSU Mechanical Engineering Assistant Professor Yang Yang to 3D print textured microchannels to visualize acoustically-driven flow patterns in complex channels.
This phase of experimentation will provide a means to validate the discoveries found in the simulation research.